What is Hydrogen Embrittlement? (HE)
Hydrogen Embrittlement, a form of Stress Corrosion Cracking (SCC), occurs when materials become brittle as a result of the introduction and diffusion of hydrogen into the metals. The degree of embrittlement is influenced both by the amount of hydrogen absorbed and the microstructure of the material itself. Microstructures which bestow high strength, often monitored by hardness level, or having specific distributions of grain boundary particles or inclusions, can result in increased susceptibility to embrittlement.
The phenomenon usually becomes significant when it leads to cracking. This happens when sufficient stress is applied to a hydrogen-embrittled object. Such stress states can be caused both by the presence of residual stresses, associated fabrication operations such as forming and welding, and applied service stresses. The severity of hydrogen embrittlement is a function of temperature: most metals are relatively immune to hydrogen embrittlement, above approximately 150°C.
What causes Hydrogen Embrittlement (HE)
Hydrogen embrittlement is a complex process involving a number of distinct contributing micro-mechanisms, not all of which need to be present in failure modes. The mechanisms include the formation of brittle hydrides, the creation of voids that can lead to high-pressure bubbles, enhanced decohesion at internal surfaces and localised plasticity at crack tips that assist in the propagation of cracks. There is a great variety of mechanisms that have been proposed and investigated as to the cause of brittleness once diffusible hydrogen has been dissolved into the metal. In recent years, it has become widely accepted that HE is a complex, material and environmental dependent process, so that no single mechanism applies exclusively. Below you’ll find some of these mechanisms:
Internal pressure: At high hydrogen concentrations, absorbed hydrogen species recombine in voids to form hydrogen molecules (H2), creating pressure from within the metal. This pressure can increase to levels where cracks form, commonly designated hydrogen-induced cracking (HIC), as well as blisters forming on the specimen surface, designated hydrogen-induced blistering. These effects can reduce ductility and tensile strength.
Hydrogen enhanced localised plasticity (HELP): Hydrogen increases the nucleation and movement of dislocations at a crack tip. HELP results in crack propagation by localised ductile failure at the crack tip with less deformation occurring in the surrounding material, which gives a brittle appearance to the fracture.
Hydrogen decreased dislocation emission: Molecular dynamics simulations reveal a ductile-to-brittle transition caused by the suppression of dislocation emission at the crack tip by dissolved hydrogen. This prevents the crack tip rounding-off, so the sharp crack then leads to brittle-cleavage failure.
Hydrogen enhanced decohesion (HEDE): Interstitial hydrogen lowers the stress required for metal atoms to fracture apart. HEDE can only occur when the local concentration of hydrogen is high, such as due to the increased hydrogen solubility in the tensile stress field at a crack tip, at stress concentrators, or in the tension field of edge dislocations.
Metal hydride formation: The formation of brittle hydrides with the parent material allows cracks to propagate in a brittle fashion. This is particularly a problem with vanadium alloys, but most structural alloys do not easily form hydrides.
Phase transformations: Hydrogen can induce phase transformations in some materials, and the new phase may be less ductile.
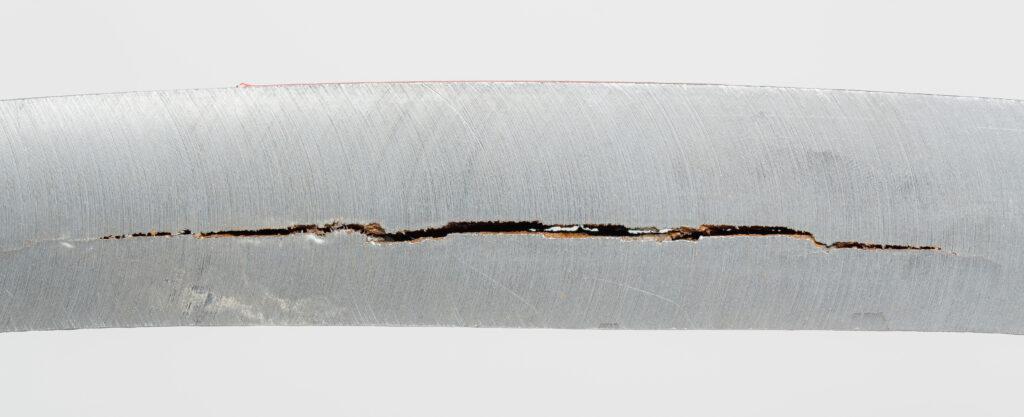
How can we prevent Hydrogen Embrittlement? (HE)
High-strength steels with tensile strength greater than about 145 ksi (1000 MPa) are the alloys most vulnerable to hydrogen embrittlement. Exposure to hydrogen occurs during surface finishing process steps such as acid pickling and electroplating and during service if the steel is exposed to acids or if corrosion occurs.
Proper material selection is essential. For example, increasing the Ni percentage and decreasing the C percentage in the material reduces the chance of hydrogen embrittlement. The addition of Ti also helps. These atoms occupy critical locations in the crystal structure of austenitic stainless steel. In other words, the added elements stabilize the desired microstructure. For safe use with hydrogen, a minimum nickel content of 10% is often maintained.
Further steps that can be taken to avoid hydrogen embrittlement include reducing hydrogen expure and baking after electroplating or other processes that lead to hydrogen absorption. Hydrogen embrittlement of electroplated components can be prevented by baking them at 375 to 430 °F (190 to 220°C) within a few hours after the electroplating process. During baking, the hydrogen diffuses out of the metal.
For applications where there will be hydrogen absorption while a component is in service, the use of lower strength steels and reduction of residual and applied stress are ways to avoid fracture due to hydrogen embrittlement.
In addition to choosing the best steel grade, the employed welding technique also affects whether or not hydrogen embrittlement occurs. By making the wrong choice of material and alloy, sensitization can occur. Sensitization is the formation of carbides at the crystal boundaries of the material during welding.
Fortunately, sensitization is preventable by using stainless steel with a low carbon percentage (<0.03%), such as stainless steel 316L or 1.4404. The addition of Ti to the alloy, as in stainless 316Ti or 1.4571, is also an excellent method to prevent this problem.
Hydrogen-Induced Cracking (HIC) & Wet Hydrogen Sulfide Damage (H2S)
Hydrogen Induced Cracking (HIC) is a form of Hydrogen Embrittlement that occurs when corrosion from acids like wet hydrogen sulfide and hydrofluoric acid cause atomic hydrogen to penetrate hardened or higher strength steels and cause stress cracking. This penetration can lead to the brittle fracture of materials that are normally ductile when hydrogen happens to be present in their environment.
How fast the hydrogen penetrates into the metal depends on the temperature and pressure of the atmosphere in which the component is located. Hydrogen stress cracking can affect several different types of metals such as high strength structural, carbon, and low alloy steels, along with titanium, nickel, and aluminium alloys. Ferritic stainless steels, on the other hand, tend to be fairly resistant to hydrogen stress cracking due to their comparatively low hardness.
Zones of high hardness and areas where steels and weldments contain high amounts of residual hardening elements are more susceptible to hydrogen stress cracking. If a component already has a crack, it is possible for hydrogen stress cracking to begin at the already existing crack.
Hydrogen-induced cracking (HIC), a type of wet hydrogen sulfide (H2S) damage, is a condition commonly observed in the refining, petrochemical, chemical, and gas sectors.
If a certain level of hydrogen sulfide is detected in carbon steel, low-alloy, or other high-strength steels, it’s deemed a sour service environment. This denotes its risk for wet H2S damage, including hydrogen blistering, stress-oriented hydrogen-induced cracking, and sulfide stress cracking.
Want to learn more? Contact us below: